Effects of Antibiotics on Animal Feed
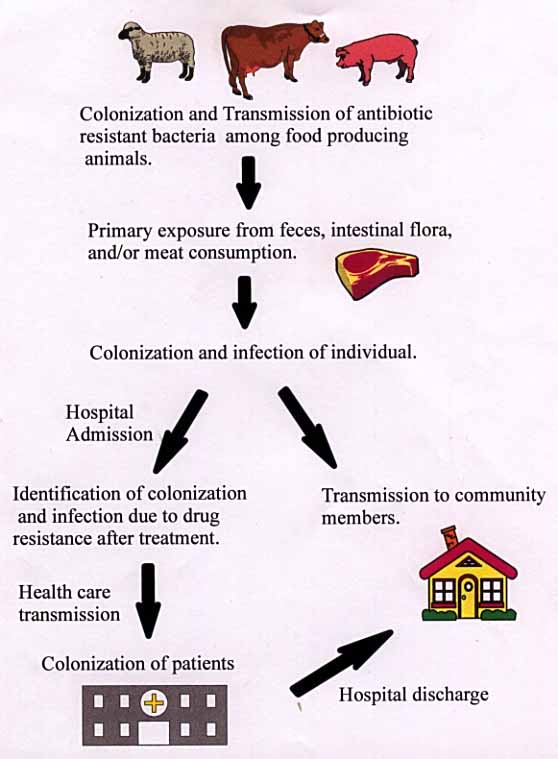
Antibiotics have been used in animal feed for about 50 years ever since
the discovery not only as an anti-microbial agent, but also as a
growth-promoting agent and improvement in performance. Tetracyclines,
penicillin, streptomycin and bactrican soon began to be common additives
in feed for livestock and poultry. Currently, the following antibiotics
are used in livestock and poultry feed: chlortetracycline, procaine
penicillin, oxytetracycline, tylosin, bacitracin, neomycin sulfate,
streptomycin, erythromycin, linomycin, oleandomycin, virginamycin, and
bambermycins. In addition to these antibiotics, which are of microbial
origin, there are other chemically synthesized antimicrobial agents that
are also sometimes used in animal feeds. These include three major
classes of compounds: arsenical, nito-furan, and sulfa compounds.
Arsenical compounds include arsanilic acid, 3-nitro-4-hydroxy
phenylarsonic acid, and sodium arsanilate; nitro-furan compounds include
furazolidone and nitro-furazone; sulfamethazine, sulfathiazole, and
sulfaquinoxaline. Other chemicals are also used as antiprotozoal agents
to prevent coccidiosis and histomaniasis in chickens and turkeys.
Antibiotics are used regularly in animal feed at a rate of 2 to 50 grams
per ton for improved performance in the animals. The reasons include a
more efficient conversion of feed to animal products, an increased growth
rate and a lower morbidity/mortality rate in general. The levels of
antibiotics are often increased to 50-200 grams/ton or more when specific
diseases are being targeted as when the spread of a particular disease is
rampant. The levels are also increased in times of stress. This
increased amount is often decreased when the threat of a disease is
gone.
The benefits of antibiotics in animal feed include increasing efficiency
and growth rate, treating clinically sick animals and
preventing or reducing the incidence of infectious disease. By far the
major use of antibiotics among these, however, is
increased efficiency, i.e. a more efficient conversion of feed to animal
products, and an improved growth rate. In chicken feed,
for example, tetracycline and penicillin show substantial improvement in
egg production, feed efficiency and hatchability, but no
significant effect on mortality. Chlorotetracycline, oxytetracyclin and
penicillin also show an improved growth rate, but little
effect on mortality. Antibiotics in animal feed, in general, are used
regularly for increased efficiency and growth rate than to
combat specific diseases.
After animals have been fed antibiotics over a period of time, they retain
the strains of bacteria which are resistant to antibiotics. These
bacteria proliferate in the animal. Through interaction, the resistant
bacteria are transmitted to the other animals, thus forming a colonization
of antibiotic resistant bacteria. The bacteria flourish in the intestinal
flora of the animal, as well as, in the muscle. As a result, the feces of
the animal often contain the resistant bacteria. Transfer of the bacteria
from animal to human is possible through many practices.
The primary exposure of humans to resistant bacteria occurs in farms and
slaughterhouses. Humans clean the feces, which contain the bacteria, of
the animals on farms. During the cleaning process, humans may get
bacteria on their body and hands. If the body or hands are not properly
cleaned, the bacteria could be ingested by the person. Likewise, in
slaughterhouses, during slaughter, the intestine is severed. Resistant
bacteria are exposed to slaughterhouse workers, which could get the
bacteria on their bodies and hands. Transmission occurs when the bacteria
is ingested. Along with the previous sources of contamination, humans can
get infected by eating meat from animals with resistant bacteria. Even
though cooking reduces the survival of the bacteria, some may still
survive and infect the human. For example, 1983, 18 people in four
midwestern states developed multi-drug resistant Salmonella food poisoning
after eating beef from cows fed antibiotics (1).
After initial transmission and infection to humans, the transmission to
other humans has a couple paths. Transmission can take place through the
many mediums (aerosol, physical contact, and bodily fluids) of human
contact in the community. An infected individual may also be admitted to
a hospital for treatment. Treatment may not work in drug resistant
bacteria, therefore, identifying a drug resistant infection. Bacteria is
transmitted to other patients via the hospital environment or health care
worker=s hands. After transmission, the bacteria will colonize in several
of the patients. Colonization in other patients with other resistant
bacteria can produce bacteria with multi-drug resistance. Once the
patients recover, they are discharged into the community. These patients
could potentially infect several community members. Multiple infection
could potentially produce a supergerm which is resistant to many drugs due
to resistance sharing between bacteria.
In most cases, the drug resistance genes of bacteria are carried on
plasmids (specifically, the R or resistance plasmid), which
replicate separately from the cell’s circular DNA. These plasmids can be
passed from cell to cell, allowing for a drug resistance
to be passed to a large group of bacteria and to different types of
bacteria. These resistance genes are also carried on
transposons, which allow for these genes to move from one strand of
genetic material to another. Because of this, a cell can
receive multiple plasmids with resistance genes and then integrate all of
the resistances onto one plasmid. Some R plasmids have
as many as 8 drug resistances on them.
The development of a drug resistance is not orchestrated specifically to
counteract a drug. Rather, drug resistances arise
because of spontaneous genetic mutations within a gene sequence. By
chance, these mutations happen to produce some change
in the cell that allows for drug resistance. This mutated bacteria then
has a selective advantage over other non-resistant bacteria.
The addition of antibiotics to the environment (the host organism) then
selects for the resistant bacteria by killing off all of the
non-resistant bacteria. This allows for the resistant cells to grow and
divide, creating a large population of resistant bacteria. The
larger population then increases the likelihood that plasmid transfer will
occur to other, non-resistant bacteria of various strains.
This attained resistance has little effects on the host organism until
plasmid/resistance transfer to a particularly virulent bacteria
occurs. Then, the host is susceptible to infection from this organism
without the benefit of treatment with the antibiotic that the
bacteria is now resistant to.
There are several general methods through which a cell can become
resistance to an antibiotic. These mechanisms are:
- 1. Decreased cell permeability to the drug - the cell can change its
membrane structure so that the drug cannot enter the cell and perform its
function
- 2. Alter the drug binding/recognition site - by changing the
structure of the membrane surface, the site which previously allowed the
drug to bind to the cell can no longer do so
- 3. Chemical modification of the antibiotic - by cleaving a portion of
the molecule or adding a substituent group, the properties of the active
molecule in the antibiotic can be altered such that it is rendered
harmless to the cell
- 4. Active transport - the transport of drug molecules out of the
cell. In many cases, this is done via a drug/proton antiport system.
With this mechanism, H+ ions are pumped into the cell as drug molecules
are pumped out.
- 5. Enzyme or pathway alteration - the cell can change the pathway or
enzyme used to carry out a cell process occurs. By doing this, the cell
can bypass the enzyme that is affected and cause the drugs effects to have
no bearing on the functioning of the cell.
There are three main ways in which genetic material (in this case,
drug
resistance genes) can be exchanged between bacteria. They are as
follows:
- 1. Conjugation - a direct, cell-to-cell, contact transmission method.
The plasmid containing cell generate a small tubule that connects the two
cells (the sex pili). This tube then allows for the passage of DNA
strands between the two cells
- 2. Transformation - the absorption of "naked", free-floating DNA by a
cell. Upon the death of a bacterial cell the cell components degrade,
leaving the DNA and cell materials to disperse in the environment. If a
cell with antibiotic resistance dies and breaks down, the resistance gene
may be released into the environment and absorbed by another bacterial
cell.
- 3. Transduction - the transportation of genetic material by a
bacteriophage. When a bacteriophage infects and replicates in a cell,
some new phages may be filled with cellular genetic material, rather than
viral genetic material. In some cases, this cellular material is a
resistance gene. When the phage containing the resistance gene infects
another cell, the infected cell then gains the bacterial resistance.
The economic impact is difficult to measure, partly because extensive
searching could not turn up exact figures for employees and profits
specifically in feed additives. However, it is safe to say that in the
U.S. alone, stopping the practice of adding antibiotics would result in a
loss of millions of dollars in profits and thousands of lost jobs. It is
also worth noting that sales of antibiotics are a very profitable sector
for companies that engage in their productions.
References
- Antibiotics in Animal Feed,
Council
for Agricultural Science and Technology, 1981.
- McDonald, L. Clifford, Matthew J. Kuehnert, Fred C. Tenover, and
William
R. Jarvis (1997). "Vancomycin-Resistant Enterococci Outside the
Health-Care
Setting: Prevalence, Sources, and Public Health Implications" Centers
of
Disease Control and Prevention, Alanta, Georgia, 3.
wysiwyg://67/http://www.cdc.gov/ncidod/EID/vol3no3/mcdonald.htm
- "The Rise of Antibiotic-Resistant Infections" (1995). FDA
Consumer, 29.
http://www.fda.gov/fdac/features/795_antibiotic.html
Return to Introduction Page.